Develop tunable, developmentally appropriate models of brain disease
The only way to simulate the complex environment of the brain is, well, the brain! We use living tissue models of the brain to study how disease, injury and treatment alter brain cells, vasculature, and extracellular spaces. Specifically, we have pioneered the use of organotypic whole hemisphere (OWH) brain slices from a range of species, including rat, ferret, mouse, rabbit, and OR-obtained human samples, and can study donor age, sex-dependent and brain-region dependent responses to various stimuli. Adapting from in vitro and in vivo techniques, we have published molecular, cellular, and functional assessments in slices to study changes in the cellular and extracellular space. The range of length and time scales possible for data generated from slice models allow us to apply data science and machine learning tools to uncover biological insights that are otherwise not obvious through standard data analysis methods. These slice platforms allow high throughput live tissue imaging in the acute and chronic windows after injury, or to study aspects of neurodevelopment, while retaining the 3D cytoarchitecture and in vivo physiological function of brain cells. A single brain can provide 20-30 slices, which also reduced the number of animal lives needed to study brain injury and treatment. In addition, we have developed microfluidic model systems of the blood-brain barrier (BBB) functions to study response to stimuli of multiple brain cell types to engage in a physiologically relevant 3-dimentional architecture in the presence of flow. Current project areas and recent publications are highlighted below.
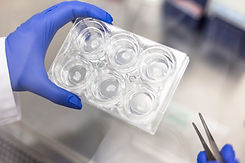
Modeling hypoxia-ischemia ex vivo
Loss of oxygen and blood flow to the brain is a common cause of mortality around birth or brain damage after birth. We have developed living brain slice models of hypoxic-ischemia injury in the rat and ferret that mimic the injury seen in vivo and clinically in extremely preterm, preterm, and term equivalent infants. We have used these brain slice models to study the effect of developmental age, sex, and severity on regional cellular response and treatment response.
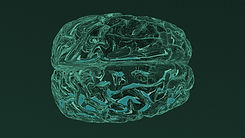
Modeling brain injury in low resource settings
There has long been a distinction in the data reported on the prevalence and associated mortality of neurological disease: incidence rates are higher and outcomes are worse in low resource settings (LRSs) compared to high resource settings (HRSs). Recent work in our lab focuses on developing ex vivo models of brain pathology in the LRS, which has more dominant white matter injury.
Microfluidic model of the blood-brain barrier (BBB)
The BBB describes the collection of endothelial cells, pericytes, astrocytes, and other cells which line vessels and capillaries in the brain. Endothelial cells also require perfusion as well as cell-cell interaction. Microfluidic modeling allows for incorporation of these cell types and flow rates that mimic blood flow in capillaries. We use a commercially available microfluidic device to investigate the effect of hypoxia and nutrient deprivation on BBB function, cell viability, and permeability in the presence of flow.
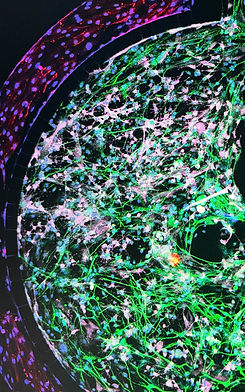
Modeling acute and traumatic brain injuries
OWH brain slice models can mimic disease hallmarks seen in acute and chronic neurological disease and be used to probe the mechanism of microenvironmental changes seen in response to disease in a regionally dependent manner. Our work in this area has investigated chronic exposure to environmental toxins that disrupt mitochondrial function, 'double hit' models with multiple stimuli, and more recently modeling blast injury or concussive forces that result in traumatic brain injury.
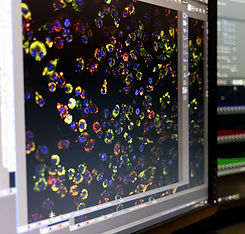
Selected recent publications:
Design principles for nanotherapeutic delivery to the brain
We have identified general design principles for engineering therapeutics for delivery to the brain, with the goal that the design principles are adaptable to any nanoparticle system. We have shown that nanoparticles of sizes ranging from 10s of nanometers to larger than 100mm can overcome the BBB when it is impaired, or when the BBB is intact, by leveraging receptor transports , osmotically induced permeability, or with external non-invasive BBB permeability strategies. Our growing evidence also demonstrates that particles reaching the brain from systemic administration should have near-neutral or anionic net surface charge, and that cationic surface charges on nanoparticles result an inability to cross even in an impaired BBB. Within the brain parenchyma, we have shown that the estimated upper limit for rapid nanoparticle transport is around 120nm, with potential for larger sizes based on results by us and others that show effective spaces in the brain can range up to 1µm in size. We have also identified the importance of surface charge and functionalization in increasing nanoparticle penetration in the brain, with neutral or anionic net surface charges imparted by hydroxyl, methoxy, or carboxyl groups driving maximum diffusive ability in the brain. Thus far, we have applied these general design criteria to nanoparticles made from polymer, quantum dot, cellulose, peptoid, dendrimer, or extracellular vesicle materials. Current project areas and recent publications are highlighted below.​
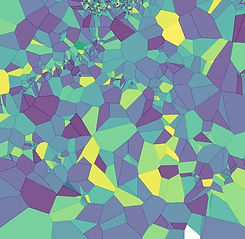
Analyzing diffusion in the brain
Through application of multiple particle tracking, we can measure the real-time x-y displacement of thousands of individual fluorescent probes in the living brain. We can quantify Brownian motion and non-Brownian motion to determine what physical and chemical properties impact the ability of a molecule or particle to penetrate within the brain parenchyma. Our current efforts in this space aim to decouple different physical and chemical properties from each other to determine which properties are the most significant contributors to a particle's ability to penetrate within the brain microenvironment.

Formulation parameters impact brain fate
In formulating nanoparticles, often times agents such as stabilizers or emulsifiers are used. These are identified by the FDA as generally regarded as safe (GRAS) and are incorporated in the final formulation for many pharmaceutical formulations, including nanoparticles, in very small quantities (<0.1% by weight). We have shown that the type of emulsifier or stabilizer can influence particle distribution in the body, uptake in the brain, and distribution and cellular localization in the brain. Our ongoing work analyzes how administration route and developmental age alter surface presentation on a nanoparticle and influence cell fate in the brain.
Nanoparticle agnostic analysis
In studying governing design principles that impact nanoparticle delivery to the brain, we have sought to be nanoparticle agnostic. We have worked with polymer, quantum dot, cellulose, peptoid, and dendrimer nanoparticles, and extracellular vesicles (a biologically produced nanoparticle). These particles can be achieve cell specific uptake in the brain if they are less than 100nm in one dimension, near-neutral or net-negative in surface charge, surface functionalized with hydroxyl, methoxy, or carboxyl groups, and have a non-hydrophobic surface. We are currently exploring the role of nanoparticle shape, architecture, and rigidity on brain delivery for different nanoparticles.
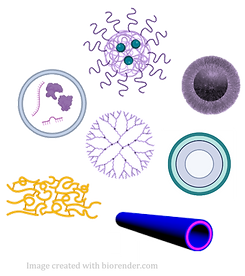

Quantifying and predicting nanoparticle fate
Although brain cell-nanoparticle interactions have been well-studied, contradictory findings for individual cell-nanoparticle interactions persist, likely due to variability of models used in each study, which can have differing transport barriers that alter nanoparticle fate. We use in vitro, ex vivo, and in vivo models to quantify nanoparticle fate in the brain. In addition, our analysis of nanoparticle behavior in the brain is data rich. We have leveraged data science and machine learning tools applied to our nanoparticle diffusion data to distinguish nanoparticle properties in living tissue, such as size and protein adsorption, and predict local viscosity and cell internalization.
Selected recent publications:
Evaluating neurotherapeutics for improving brain health in newborns and children
Therapies intended for use in children can take up to 7 years longer to go from the first clinical trial in adults to the first trial in children; often, many approved adult therapeutics are used off-label for children. There is a significant gap in technological development for the neonatal and pediatric populations, particularly in technology that focuses on improving therapeutic outcomes for children and newborns with a range of conditions. Our research seeks to develop and evaluate therapeutic delivery systems for newborns and children, who have unique physiologies compared to adults. We focus specifically on engineering therapeutics that mitigate or attenuate ongoing injury in the brain, with the goal of improving neurological function and quality of life across the lifespan.